Green Gas the Cinderella of energy - by Bill Powell
Green Gas the Cinderella of energy
by Bill Powell bill@vts16.fastmail.fm
17/10/2016 (x) indicates a Note.
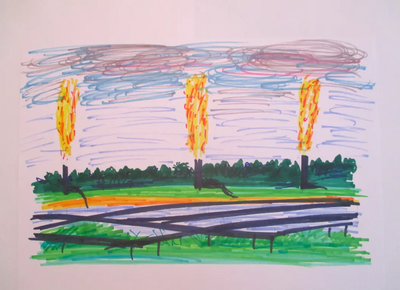
BACKGROUND Former energy minister Ed Davey told the Liberal Democrat 2016 Spring conference that we'd continue to need gas for heating until at least 2030.
a) I and only two other participants in the LibDem working party on energy policy had a professional engineering background. There we found that the consensus was that all UK premises could be raised to a 'Passivhaus' standard so there would be minimal need for heating. One of us suggested that there were 30 million premises (23 million residential) that would need £35,000 or so spent on each to achieve that. Multiply and the result is £1 trillion. This would not be government but private expenditure. He suggested such expenditure was unrealistic. But we were told that we were contributing to a political not an engineering document.
Another unspoken assumption looks to an 'all-electric' future for heat and transport as well as electric power. Energy delivered to a visible 13A socket can do all sorts of things that gas cannot do as efficiently or cannot do at all. What is invisible is the heat from gas which is taken for granted except when you return from a winter holiday and find your house freezing cold.
The late Sir David MacKay in his book 'without the Hot Air' used a factor of 2.5x to express the relative 'value' of the two forms of energy. Specialists would say that the 'exergy' of the two forms of energy are different even though the killowatt hours (or PetaJoules, etc) are the same.
He brought much sense to confused journalism and politics, but like our working party, he was unrealistic about the implications for the public. In the case of heating he showed how electric heat pumps could deliver 2.5x or more heat than the same kWh of gas energy sent to a gas boiler. The problem is that people already have very efficient gas boilers and pay at least 2.5x less for their gas than for their electricity. Telling them to discard those boilers and buy expensive electricity and heat pumps instead (and change all their radiators to accept a lower water temperature heating system) gains next to nothing on running costs. It will not be popular.
This huge capital expense by users could be avoided by simply reducing the carbon footprint of our gas supplies. 'Green Gas' is truly the Cinderella approach that receives very little attention.
b) An even bigger problem is disbelief that sufficient gas can be made 'green' without starving us all or cutting down the world's forests. Yet there are already huge quantities of domestic, municipal, farm and forest waste (a) in Britain that could replace 40% of the natural gas (NG) we use for heating. In many EU countries there are even greater forest wastes which could (b) provide enough energy for not only heat but for transport and electricity also.
This waste contains stored energy which can be converted to and stored as fuel gases to provide dispatchable heat (or electricity). Though wind and sun do provide electricity directly, they are not reliable. It may be that some cost effective battery technology will enable sufficient electricity to be stored (c) to balance the electricity grid, but there could be enough waste material to provide the fuel needed to do this as well as provide some 'green-gas' for heating.
There is also fear of change especially if the green gas is hydrogen. Even MacKay wrote that hydrogen in your fuel cell car would all escape the gas cylinder within a week. I, through a Birmingham professor, managed to get that changed in the on-line edition of his book (d). Town gas was dangerous not because it was 50% hydrogen, but because it contained 20% odourless but poisonous carbon monoxide (CO). Likewise today it is CO poisoning from badly installed NG boilers that are the greatest danger. As to the danger of ignition, both NG and hydrogen can form a combustible mix with air, but explosion should be less likely in the case of hydrogen as it disperses so much faster than NG.
c) Wood burning stoves are becoming popular as people understand that the CO2 they release is absorbed again as new trees grow. Therefore wood should be a carbon neutral source of energy.
Yet the timescale between burning and regrowth does matter because between these events there will be an increase in CO2 levels. Trees take 15 to 20 years to mature. What matters is the annual yield per hectare. This is usually expressed as 'oven dried tonnes' (odt) of biomass. Each odt contains about 4,600 kWh energy. Miscanthus, which can be easily harvested, takes about 4 years to reach full production and can yield up to 25 odt/ha/year, though 17 odt may be a more cautious figure. Willow can also be grown in the cooler parts of the UK. In equatorial areas yields can be much higher. For example bamboo yields about 4x as much per year. Cacti such as agave or prickly pear grown in semi-desert areas can produce about half that of bamboo.
For centuries we have bred plants to increase food rather than fuel yields. If we returned to growing the wheat grown prior to WW2 we would have both food and fuel. There is also potential for double cropping where a fuel and food crop follow one another in the same year.
In these ways sustainable fuel gases could be produced in a very short cycle. The process would be carbon neutral. However, the process of producing fuel gas also delivers captured CO2.
If this is sequestered as proposed for fossil fuels the cycle becomes 'carbon negative' actually removing CO2 from the atmosphere. This is BECCS (bio-energy with carbon capture & storage) which is at long last exciting politicians. Recent modelling suggests that the CO2 reduction targets agreed in Paris cannot be met without carbon negative technology such as this. (r)
There is a simpler yet even more important way to make the process carbon negative, and that is to sacrifice some of the energy contained in the carbon content of the feedstock to produce bio-char as a by product. Instead of burning it at a barbecue it can be incorporated into fertilisers where it improves the soil structure. This is not the place to go into details here except to note that this 'terra preta' is found along the banks of the Amazon where a lost civilisation did just this to prevent the river washing nutrients out into the Atlantic. The process is in effect the reverse of fossil fuel mining. The biochar remains in the earth permanently.(e)
d) There are three ways to produce 'Green-Gas'
- Electrolyse excess wind and solar power to produce hydrogen. The process should be at least 70% efficient and can operate under pressure making the gas easier to store or deliver. ITM Power of Sheffield (I declare a very small interest) are selling electrolysers most notably in Germany where there is frequently excess wind and solar energy. We too are entering more frequent periods of excess when otherwise we have to 'throw this energy away'. This hydrogen is very pure and is therefore ideal as fuel for fuel cell vehicles. It can also be blended with the NG sent to our homes. The conclusions of the EU NaturalHy study (f) encouraged initial blending of hydrogen up to 20% by volume in gas supplies, (some EU countries have so far approved blends up to 5%).
- Anaerobic Digestion (AD) is at last becoming more wide spread. The gas this produces is roughly 50% methane. (NG in our gas mains is nearly pure methane) Some processing is therefore needed to purify the methane in order to blend it with the NG in our gas mains. The cost of doing this has been a disincentive to its production. The AD feedstock has to be 'nutritious green waste' of farm or human origin. The process takes ten or more days and leaves a 'digestate' that is generally spread on farm land to return many nutrients such as phosphorous, etc. to the land. The digestate also contains 'stringy and woody' material that contains about the half the energy of the original feedstock. The bugs and beetles in farm soil thrive on that energy in this material converting it to CO2. Landfill sites have been an accidental source of this type of methane gas and until we built so many wind turbines during the 2010-15 coalition it was our largest source of 'renewable energy'. Sewage farms produce this gas but it is usually used on-site because of public perceptions that sending it elsewhere might spread disease. That's nonsense! The digestate from sewage farms is now spread on farmland. But some of the federal states of Germany have forbidden this out of similar fears. This could lead to the digestate being either incinerated or processed by thermal gasification to release the 50% energy content that AD fails to extract.
- Thermal gasification goes back to 1793 when William Murdoch installed gas lighting into his home. Feedstock can be coal or biomass and releases virtually all the energy of the feedstock. The processes can be observed in an open wood fire. First heat drives flammable 'pyrolysis' gases out of the wood which burn in air. These gases include large molecules which would condense to oil and tar, as happens on the walls of people's kitchen ovens. Later and at higher temperature the wood blackens as its structure breaks down releasing further fuel gases and leaving the charcoal skeleton of the wood to be burned last. Ash falls the bottom of the fire. Finally this biochar burns. Instead of an open fire these processes are totally enclosed in a chemical reactor. The pyrolysis gas is diluted by the high proportion of nitrogen present in the air used as the oxygen supply, but this gas can be used for heating, gas lighting or to run a motor generator for electricity. The danger is that tar will condense and clog the pipes. Temperatures of 850 C or more are used to break down large pyrolysis molecules into small ones of hydrogen and carbon monoxide which is known as 'syngas', short for 'synthesis gas'. This refers to its use to produce a huge range of chemical products such as plastics, ammonia fertiliser, liquid fuels and 'synthetic natural gas' (SNG). There are further details depending on the purity of the syngas needed. They include ash and CO2 separation, the use of oxygen instead of air to avoid the dilution of the gas with nitrogen, and finally mixing steam with the supply of oxygen. The oxygen reaction causes the temperature to rise, but steam does the opposite. In doing so the steam molecules break down into hydrogen and oxygen. The result is that instead of excess heat, the process delivers more hydrogen in the syngas. The process becomes more efficient at large scale and under pressure. It takes only about 20 minutes to decompose biomass into syngas. When the feedstock includes poisonous content (even medical waste), the temperature is raised even further (1,200 C or more) so that the ash melts into glass-like 'slag', which entombs any solid poisons. On the other hand ash from clean agricultural waste can be returned to the land returning valuable nutrients to the soil. Biochar can also be extracted before it too is fully oxidised. Town gas was produced from coal in this way. Hitler's empire lacked oil and oil embargoes on S Africa led both to build huge gasifiers for oil from coal installations. SASOL now lies at the centre a huge S African chemical industry. Ordinary citizens in the Third Reich turned to the forests for fuel and built small biomass gasifiers. Sometimes these were actually built into the car or van. When, as a weapon, the Arabs cut off oil supplies to the West the US government developed and issued plans to fit emergency gasifiers to farmers' tractors. I have the plans! Small gasifiers are being introduced into rural India and Africa by NGOs to provide village electricity.
e) The small pyrolysis gasifiers in Africa and India generally have an output of a few tens of kW up to 1 MW. They are invariably used to drive an electricity genset, and this capacity refers to the electrical not the fuel gas output which is much higher. A good genset may have an efficiency of 35% in converting fuel gas to electric power, and even a small gasifier can have an efficiency of 70% in converting biomass energy to fuel gas. Together the overall efficiency to electricity is only about 25%. Given that 1 tonne of biomass contains about 4,500 kWh you could work out how many tonnes / day are required to produce 1 MW output, and how many hectares of miscanthus or bamboo would be needed to supply that much. This also shows that in terms of kWh three times as much gas as electric energy is being produced. But beware of fuel efficiencies. They can be defined in different but valid ways. (g)
Engineers who have worked with Town Gas are convinced that the large coal fired gasifiers they knew could be converted to biomass to produce huge quantities of 'green-gas' to replace the NG we now distribute. They envisage 50 MW and greater size (gas not electric MW) which would serve at least a market town. (Drax power station shows how this is being done for electricity. Drax can produce about as much electricity as the proposed Hinkley Point nuclear power station.)
Just as coal was stockpiled for the winter, so can biomass. The gas produced can also be stored and a huge amount is present in the gas pipes themselves. Indeed the variations in the demand for gas are taken up by energy stored in the pipes. This is unlike the electric grid which stores no energy and so requires huge amounts of standby generating capacity ('spinning reserve') to cope with variations in demand.
The capital cost of building these large gasifiers would be much lower than building a power station. A typical figure quoted for the cost of an Integrated Gasification Combined Cycle (IGCC) power station for CCS is 30% higher than a normal CO2 emitting gas fired power station. A coal fired IGCC power station consists of a gasifier to produce separate streams of CO2 for sequestration and hydrogen to feed the gas turbine generators. Leave out the electrical generation and you have the cost of the gasifier. And since three times as much energy can be delivered to end users as gas than as electricity the cost of gas per MWh should be only a third the cost of electricity.
f) It was Greg Barker who remarked 'Big boys build big power stations'. It's as if leaders need to build monuments to themselves. Our grandfathers built battleships. As Chris Huhne took up the reins at DECC he remarked that 'This is not an energy department, it's a nuclear department'. James Lovelock remarks in his book 'A rough ride to the future' that big companies bring huge funds to lobby for huge contracts that are underwritten by tax payer subsidies. These appear to be the reasons that greening our gas supplies is so overlooked. Where is the global company interested in a project one third the value of building a power station and then to selling its product at one third the price?
These appear to be the reasons why 'green-gas' remains the Cinderella option for providing sustainable carbon neutral energy.
THE BIG PICTURE
A) Nuclear power supplies about 20% of our electric power, but that is only 8% of our total primary energy yet it receives disproportionate attention. A nuclear power station is also a thermal power station and, like gas and coal fired stations, produces huge amounts of heat which is usually released into the sea where it changes the marine ecology. If near a town this heat could be piped to homes as heating.
The economic case for nuclear is that it produces energy 24/7. Spreading the cost of capital over 365 days is much more favourable than the many fewer hours in a year that wind and solar produce energy. The output of nuclear power stations cannot be varied much so it cannot share in the role of fossil fuel power stations whose output can be varied to balance the demand. Suppliers of those complain that consigning them the role of balancing the grid means they operate for many fewer hours than intended which effectively raises the cost of their capital per MWh so much that they can become uneconomic.
All the attention on BIG nuclear distracts attention from other nuclear options such as small breeder and thorium reactors. When a nuclear submarine pulls into harbour the population does not leave in panic. There is therefore an option of installing small nuclear reactors in underground silos near each town. This could be near enough to also use their waste heat. Every big nuclear power station is virtually a 'one off' whereas such small nuclear stations could be mass produced leading to much lower capital cost. These points do not mean I favour nuclear over biomass as a primary source of energy. But it suggests that 'small nuclear' deserves much more attention. Breeder reactors can include much of our nuclear waste in their fuel. The waste from thorium reactors decomposes in historic periods as opposed to geological periods for uranium. I do not regard nuclear as truly 'sustainable' as we would need to discover much more uranium if the world were to generate all its electric power this way. Thorium is said to be much more plentiful, but I fear that protecting thorium nuclear waste for even historic periods is a problem we have no answer to.
B) The goal must not only be zero emission, it must also be sustainable and affordable.
Improving efficiency in the way we use energy helps greatly because that reduces the primary energy we need helping all three goals. So we need better insulated buildings, lower fuel consumption and hybrid and electric cars, etc.
Energy is lost, invariably to heat where there is no use for it, at every sequential process: generation, storage, delivery and use. Wind and sun can be electrolysed with 75% or more efficiency, and can be delivered via the gas grid to a boiler with very little loss, but if it is stored and then used to generate electricity the latter process is only 55% or so efficient. Multiplying shows an overall efficiency from electricity and back again of only 41% unless waste heat can be captured in which case the CHP process is about 85% efficient delivering 64% overall.
Another example is fuel gas from biomass. That process can be about 85% efficient, and that will be achieved if sent directly over the local gas grid to a boiler. But if, as discussed above, it is used to produce electricity the efficiency is far lower unless the waste heat is used (Combined Heat & Power).
There are generally many other steps, each contributing a low loss, but accumulating into a more substantial loss. For fuel gas from biomass the gas must go through various stages of clean up and CO2 separation as well as conversion into either hydrogen or SNG and possible pressurisation for use in vehicles. In the case of electricity the wires and transformers contribute an average of 6% loss, but this loss is a square law, so heavy use, such as on a cold winter night when people bring out fan heaters, it might increase those losses to 20%. Storage in batteries is also lossy, and varies greatly with the type of battery. Even pumped storage hydro-electric systems lose efficiency.
The underlying messages are to keep things as simple as possible; when waste heat is produced, as in electricity generation, make use for it; do the simple things like loft insulation, but beware of idealists who are blind to cost and argue for all buildings to become zero carbon or all electric.
C) Although renewable electricity is being produced directly from wind and sun and power from the sea is joining them, none produce power more than 40% of the time. The electric grid is like a bicycle chain. It does not store energy. All it can do is average diverse sources of energy.
Contrast this with nuclear which generates power all the time or with thermal power stations which can generate power on demand, including all the time. Security is improved by combining diverse sources of energy and installing several times their 'nameplate' capacity. But costs escalate because not only must excess capacity be installed, so do infrastructure costs as each source requires its own contribution infrastructure. Bio-fueled electricity generation is the only truly sustainable option that does not suffer this cost multiplication.
Britain probably has the most plentiful wind and sea energy in Europe, and as the contribution from the sea increases the process of the power averaging on the grid will become more favourable. It may then be possible to 'keep the lights on' without thermal generation, but it still looks risky and costly compared to using fuels which include 'green gas'.
D) The EU, LibDems and Parliament set target dates to reduce our overall carbon footprint for power, transport and heat with specific targets for power and transport. No specific dates appear to have been set for gas supplies. Surely target dates should be set for gas?
E) The direct costs of the energy we use is on our fuel bill, but there are also 'external costs' such as the effects on health. A recent IMF report put these at £406 p.a. per person (h). This is usually ignored in UK, but in Germany it is used to justify high levels of subsidy to reduce these external costs. All the cost of developing future clean and sustainable energy is being allocated to our fuel bills. It is not clear why it is not taken out of general taxation. Then those subsidies could be seen in relation to 'externalities' costs such as for the NHS.
The whole thrust is to do everything through 'the market'. Every step must be seen to be profitable. If we were serious about the urgency for clean sustainable power we would take a more 'military approach' which does not expect to make a profit. We would simply go ahead as fast as possible with the most promising technologies, and very likely pursue several options in parallel.
This money minded approach is now used even by the engineer in a power station who no longer watches how much spare capacity he has but instead watches the price of energy going up and down minute by minute. He could just as well leave out the £££ and look directly at the MW head room of his power station before it becomes overloaded.
PRIORITIES
i) Do not buy big new nuclear power stations. They are too expensive and will divert investment from better and lower cost alternatives. Buy just enough gas thermal power stations to 'keep the lights on'. Answer any outcry that these emit CO2 with the promise to convert them to run on 'green-gas'.
ii) Drop plans to electrify rail by overhead wires. They are unreliable and costly not only in electrical infrastructure but also in the need to raise bridges. Instead provide the electricity from fuel cells on board the train. This could be developed faster than HS2. (And why not HSUK (i) which is appears to be a much better buy than HS2?)
There are already times when electricity demand is weak and there is excess wind supply. This can be electrolysed to very pure hydrogen at train stations and for fuel cell cars at service stations. We have UK suppliers for both the electrolysers and fuel cells. What we need is the commitment to do it.
iii) Develop 'Green Gas' as described in d) and e) above. Anaerobic digestion (AD) is gradually becoming more widespread, but subsidy is needed to purify it and connect it to the gas main. The cost of doing so should not be borne by the farmer. This would accelerate its introduction in the UK and drive down its cost.
Despite its long history thermal gasification is less well known. Small scale pyrolysis gasifiers are used with more woody material to generate electricity from forest 'thinnings' and also from farm waste in India and Africa for rural electrification. In some cases the gasifiers are designed to produce biochar as well which can be sold into emerging cities for cooking or preferably to return to the land as a long term soil improver. This and AD are priorities in emerging countries and should receive carbon credits from developed countries.
(Pyrolysis gas is a complex mixture of gases dependent on the feed stock and gasification conditions. This is attracting a huge academic research in the hope of isolating useful quantities of liquid fuel. This effort should be better employed introducing a 'green gas' economy.)
Waste recycling sites now produce three well separated streams of feedstock. That which is very wet is most suitable for AD. The other two are either contaminated or 'clean'. Both can be gasified to produce syngas.
Contaminated waste has been gasified at a UK designed plant at Avonmouth since 2001 (j). It operates at 1,250 C at which temperature the ash melts trapping contamination into molten 'slag'. That company failed at the time of the 2008 financial crisis. A 'plasma' gasifier from Japan which operates at about 5,000 C is undergoing trials on Teesside. I do not have figures for the efficiency of either, but am concerned that the efficiency of a plasma gasifier may be low due to the unnecessarily high temperature and its use of electricity to produce the plasma. Electric power, as discussed above, is both expensive and valuable. These are by no means the only 'slagging' gasifiers. Chris Hodrien proposes a very large one based on the conversion of the huge Lurgi coal gasifier to biomass(k).
At 850 C the ash does not melt, and when produced from clean waste contains valuable plant nutrients. The process can, for a small loss of efficiency, be adjusted to deliver biochar with the ash as a long term soil conditioner. The char remains in the ground making this a form of permanent carbon capture and hence 'carbon negative'. See (l) for such a proposal.
Both slagging and non-slagging large scale gasifiers should be developed to produce 'green' syngas. When properly designed the tar content of both should be very low even before further processing into SNG or hydrogen.
iv) Put 'Green Gas' into the gas grid. The most obvious 'green gas' is SNG produced either from anaerobic digestion, or by 'methenation' of syngas. Gas storage, the gas grid, users' boilers, generators and metering need hardly any change.
Hydrogen however would be better. No carbon would reach end users. All would be captured centrally making it easy to sequester all the carbon content. No poisonous carbon monoxide would be produced at end users. Methenation of syngas to produce SNG produces waste heat but hydrogen production does not. Hydrogen production should therefore be more efficient.
User appliances and metering had to be changed when NG replaced town gas (which contained 50% hydrogen). Such changes would have to be reversed to change to hydrogen. That could be done area by area until the whole country was converted (q).
Many argue instead for the gradual introduction of hydrogen by blending it with NG (or SNG) (f). A difficulty remains in that gas meters read the volume of gas not the energy delivered. A supplier would have to know the calorific value of the gas it had supplied to calculate consumers' bills. That could vary with the mix of the gases in the blend but knowing it should not be an insurmountable obstacle.
v) Accelerate the introduction of fuel cells Electricity and heat (CHP) can be produced at end users in gen sets or fuel cells. Gen sets can cope with a wider variety of fuels, but in comparison with fuel cells can be noisy, polluting and somewhat less efficient.
There are however two classes of fuel cells. High temperature fuel cells can run on SNG but they cannot be turned on and off as quickly as low temperature varieties which are very flexible. This makes low temperature types ideal for use in vehicles where power demand varies with driving conditions. They also have better proven lifetimes.
Both classes can be 'poisoned' by contaminated fuel. Low temperature varieties require very pure hydrogen. This is a further factor in the choice of 'green gas' to be put into the gas grid.
vi) Migrate from Central to Distributed Generation. The generation of electricity from fuels produces 'waste' heat which need not be wasted if there is a use for the heat as well as the electricity. The net result is a reduction in fuel a customer needs for their heat and power.
Heat from thermal power stations can be delivered to end users by building a heat distribution network, but this is expensive infrastructure.
Efficient modern gas powered 'gen sets' could be installed in 'campus' situations such as hospitals, office blocks and retail arcades to deliver both heat and power (CHP) (m). The improvement in efficiency is not well enough known.
The next step toward distributed generation would be to do the same in our homes using residential fuel cells for both power and heat. Even in summer households can use the 'waste heat' for hot water.
This distributed generation not only makes use of 'waste' heat, it also reduces losses in the supply of energy. The losses in the electric grid are usually low, but the losses in the gas grid are virtually nil. Such a system would be more efficient and more responsive to demand than the use of fossil or nuclear power stations.
vii) Heating, Heat Pumps & Smart Meters. Smart meters that simply display consumption and transmit this data to the supplier achieve little apart from making redundant those who are now sent out to read our meters.
Smart meters that can turn off heating appliances during peak demand can save the country expense of a few power stations. That same energy will still be needed but can be supplied when demand abates. They are most effective where a user consumes large amounts of electricity for heat, (and perhaps to charge an electric car). The priority is therefore not for the typical UK home which is heated by gas, but those without gas and 'campus' type users where there will opportunities for smart 'load shedding'.
It was shown at the outset in section a) that discarding a gas boiler and using a heat pump driven by electricity from a power station saves little or no fuel. However a heat pump can save up to half the fuel needed when included in a CHP gen set or fuel cell system. For example a FC using 2 kWh fuel produces 1 kWh heat as well as 1 kWh power. That 1 kWh power can pump 3 kWh heat into the building. This amounts to 4 kWh heat in all from only 2 kWh green gas.
Instead of these aspects current attention is focussed on insulation. This is appropriate for new buildings but ignores the far bigger problem of our existing stock of buildings. Also little mention is made of improving heat distribution (heating from below instead of using panel radiators which act as a chimney carrying heat to the ceiling); or of better control of when, where and to what temperature to heat the rooms of a building. My experience of updating the heating of an English 'semi' illustrates some of these ideas (n).
viii)Agriculture is now a serious source of emissions. Top soils have been losing their carbon content. Deforestation and desertification are damaging micro as well as global climates. This need not be so. In fact agriculture could make the biggest contribution to halting or even reversing climate change.
Civilisation has so far concentrated on food production. There has been little effort to breed crops for energy. Biochar can be produced as a byproduct of gasification and incorporated into the soil to improve its fertility and into animal feed to reduce their emissions. Allan Savory has made controversial proposals for animal husbandry that he claims can reverse desertification (o). The Sahara Forest Project could also bring crops to desert lands (p).
ix) Deployment, Development & Research need greater support, starting not from research, but from deployment. It is a misconception that progress comes from science. Just as a nutritionist might provide a chef with ideas to make a tasty meal more nutritious, so product development starts not with science, but with the deployment of a working product that science then helps to improve. Here are a few, but not exhaustive list of technologies that appear promising:-
- The Sea: Underwater 'windmills' can capture the 'rivers in the sea', such as the gulf stream, as well as tidal flow. There is huge potential off our coasts, especially off the west coast of Scotland. Trials are already under way.
- Algae: Research is still in its infancy, and I doubt sufficient acreage can be allocated for ponds even if successful. Yet algae appear to promise much higher yields of 'green' liquid fuels than current methods. It has even been found that algae can produce syngas directly from sunlight.
- Kites and Floating Wind Turbines: These could enable additional, and possibly less expensive, ways to capture wind energy.
- Thorium and Small Scale Nuclear: These are potentially safer and lower cost than big nuclear, and some designs hold the promise of consuming our existing nuclear waste. They are already used in nuclear submarines.
- Underground Gasification: This is really a version of thermal gasification carried out by pumping oxygen (or air) and steam into a coal seam to produce gas instead of coal. That gas is fossil, not green, but advocates claim that the carbon can be separated and pumped back and sequestered as carbon dioxide into the previously gasified coal seam. On this basis energy reserves in the form of coal are much greater than could be mined by conventional methods. The technique might also be applicable to exhausted oil fields.
- Geothermal Energy: In some places such as Iceland heat from the core of the earth is close enough to the surface to drive electricity generators. In other places, including Southampton, it is possible to extract lower quality heat to heat homes and buildings. Mining technology is developing deeper and deeper mining techniques and as it does so, it will be come possible to extract geothermal energy in more and more locations.
Further Information (Starred items '*' are available from me)
(a1*) Mike Weaver (Pyreg UK): "Generation of Renewable Heat & Sustainable Bio-based Products from Biomass" (Sustainability 2011). Estimates of 'clean' waste in UK vary greatly. This looks credible: Forest 86 Mt; Agricultural 19 Mt; Domestic Gardens 12 Mt; Industrial Organic 7 Mt; Domestic Food: 6 Mt TOTAL = 130 Mt
(a2*) Chris Hodrien (Talk given to Inst Mech Eng, Leicester 9/2/16) included contaminated waste and estimated there is enough UK waste to provide 40% of the gas used for heating. Slide 36 shows co-firing of 240 Mt waste plus 60 Mt coal (or biochar).
(a3) ENERGY RESOURCE? Conservative figures for this resource could be 200 Mt p.a. among 65 million citizens = 3.1 tonne/person p.a. = 8.4 kg/person/day. Each kg contains at least 4 kWh energy giving each person 34 kWh /day from waste. D Mackay estimated that we each use 125 kWh/day (heat, power & transport). The waste resource is therefore 27% of current needs. In the text above you will see many ways in which our needs could be reduced. Yet waste is at least enough to provide the standby power needed when sun, sea and wind fail. Additional green-gas could come from: electrolysing surplus electric power, growing and importing biomass, or the use of fossil fuels with CCS as proposed for converting the gas grid in Leeds to hydrogen (q).
(b*)Karl-Heinz Tetzlaff ( www.green-gas.net/k-h ) is a commented translation of his web site. See also W H Powell "From here to Hydrogen" https://dl.dropboxusercontent.com/u/56925069/From_Here_to_Hydrogen.pdf
(c) Significant electric power cannot be stored directly. It can instead be stored as gravitational energy such as in pumped storage, or in a reversible chemical reaction, or in other indirect ways. All are subject to loss from electricity and back again. Batteries are heavy and bulky but further improvement by a factor of 3 is possible. This could increase the range of electric vehicles to compete with fuel cell and petrol. Even if these can be 'fast charged' there will still be a problem. The transfer of electrical energy to the battery is many times faster than when driving, and is further multiplied by the number of cars being charged. The provision of such huge and intermittent supply of electricity will be a formidable problem. But there is no problem filling a car with liquid or gaseous fuel.
(d) David MacKay "Without the Hot Air" https://www.withouthotair.com/
(e*)YouTube: "Bamboo, Pyrolysis, Bio-Char & Mycorrhizal Fungi: Nature's Machine to Draw Carbon Down Exponentially" Excellent summary of bamboo to carbon capture, gasification, etc.
https://www.youtube.com/watch?v=5Czs3kI8Rk4
(f*) "The NATURALHY Project: Questions and Answers" Summary of this EU study. Slides 11 onward show no problem at the low pressures which connect to end users. Steel embrittlement could be an issue in high pressure long distance pipelines.
(g) "LHV calculations assume that the water component of a combustion process is in vapor state at the end of combustion, as opposed to the higher heating value (HHV) (a.k.a.gross calorific value or gross CV) which assumes that all of the water in a combustion process is in a liquid state after a combustion process." (Wikipedia) That is they differ by the Latent Heat of boiling.
(h) "Real cost of fossil fuel subsidies revealed in IMF report" http://eandt.theiet.org/news/2015/aug/fossil-fuel-subsidies.cfm
(i) "High Speed UK" http://www.highspeeduk.co.uk/ Offers far better rail coverage of UK regions than HS2 proposals.
(j) www.letsrecycle.com/news/latest-news/avonmouth-pyrolysis-firm-baled-outby-ethos
This and other UK projects have had nowhere near the investment of €60 million that K-H Tetzlaff (b) estimated is necessary to properly develop an efficient thermal gasifier. Clearly the gasification industry and infrastructure is also very fragmented. However, compare these figures with Nuclear!
(k) Williams, Day & Hodrien: www.carboncapturejournal.com/news/low-carbongas-from-mixed-waste-biomass-and-coal-a-low-cost-route-to-ccs/3408.aspx?Category=all Rather technical summary of technical progress in large scale gasification and proposal for co-firing of biomass with coal. See also (a2)
(l) See K-H Tetzlaff (b) and (e) above
(m)www.energ-group.com/combined-heat-and-power Ener-G is one of several companies succeeding in reducing emissions and fuel consumption through CHP. See for instance Newcastle Football club.
(n) See how I reduced the carbon footprint of my house https://dl.dropboxusercontent.com/u/56925069/My_EcoHouse_5-2012.pdf
(o) Allan Savory claims that desertification can be reversed by grazing animals in a similar way to how they graze in the wild. https://en.wikipedia.org/wiki/Allan_Savory
(p) http://saharaforestproject.com
(q) There is a proposal to convert the gas supply to Leeds from NG to hydrogen as a first step to a UK hydrogen economy. www.northerngasnetworks.co.uk/wp-content/uploads/2016/07/H21-Executive-Summary-Interactive-PDF-July-2016-V2.pdf
(r) Recent carbon emission models are having to include BECCS in order to meet the Paris targets. Here are two web reports:- www.carbonbrief.org/beccs-the-story-of-climate-changes-saviour-technology and https://climatecrocks.com/2016/09/19/are-negative-emissions-the-only-pathto-2-degrees/
Bill Powell bill@vts16.fastmail.fm